Gravoturbulent Fragmentation
Contact: K. Dittrich
A very attractive way to form the kilometer sized planetesimals in the early solar system is the 'Gravoturbulent Fragmentation' of a cloud of relatively small icy and dusty objects. In a nonturbulent e.g. laminar disk sedimentation of particles leads rather to a Kelvin-Helmoltz instability driven turbulence than to a particle layer dense enough for gravitational collapse (Goldreich-Ward instability). Also a pure hit and stick scenario (Coagulation) for planetesimals fails as either the large radial drift velocity leads to a quick decay of meter sized boulders into the star or collisional velocities are large enough to grind boulders to small debris, as was shown theoretically and experimentally. Hence, Johansen et al. (2006, 2007) came up with a hybrid scenario in which turbulent concentration of centimeter to meter sized icy and dusty material leads to sufficiently large densities in which self gravity dominates over gas shear and tidal forces of the star, thus the heaps of material collapse spontaneously under their own weight into many kilometre sized planetesimals. In the proposed project we aim to investigate the physical and mineralogical consequences of this new formation scenario for the bodies in the early solar system (planetesimals, asteroids, comets, chondruls, etc.) in a qualitative as well as a quantitative way and compare it with observations.
In the figure below from Johansen et al. (2007) we give time series of the collapse of overdense seeds in the early solar system into gravitationally bound boulder clusters. The central block of four panels shows the column densities of the four different sizes of boulders (in units of the mean column density of each size) plotted independently at a time just before self-gravity is turned on. All four particle sizes have concentrated at similar locations, an important prerequisite for the subsequent gravitational collapse. The surrounding panels show a time series of total column density of solids, in the radial-azimuthal (x-y) plane of the disk, summed over all particle sizes, starting from the upper left and progressing clockwise. Values are normalized to the average value across the grid (see colour bars in upper right panel). Times are given in orbital times Torb after self-gravity is turned on. Inset in each panel is an enlargement of a square region (indicated in the main panel) centred around the Hill sphere of the most massive cluster in the simulation, represented by the white circle. These insets show the log of the column density ratio (see colour bar in upper right panel) to capture the extreme values reached. Overdense bands initially contract radially, forming thin filaments with densities high enough for a full non-axisymmetric collapse into gravitationally bound clumps to take place. As time progresses, the Hill sphere increases in radius as the clusters grow in mass by accreting boulders from the turbulent flow.
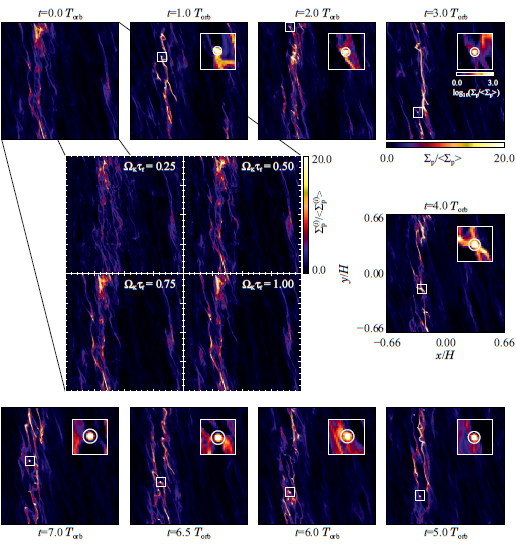
This figure from Dittrich et al. (2013) shows a snapshot of a
zonal flow
environment. The color code shows the gas surface density and the black
dots show St=1 particles (about 60 cm in size; at 5 AU in a minimum mass
solar nebula). The banded axisymmetric large-scale structure are density
bumps that are stable for several tens of orbits. They slow the radial
drift of the particles - sometimes the drift is stopped entirely. This
leads to a density enhancement of the dust particles. If such a density
enhancement becomes large enough, the particle cloud can become
gravitationally unstable and collapse into a bound planetesimal.
Streaming instability (Johansen & Youdin 2007 and Youdin & Johansen
2007) can enhance the dust density further.