How stars grow into heavyweights: Astronomers find disk around young massive star
How do stars reach masses more than 100 times the mass of the Sun? It has long been suspected that gas and dust disks circling
young stars could play a role, funneling matter onto what will become some of the most massive stars known. Now, a team
involving MPIA astronomers has for the first time detected a stable disk around one of the most massive newly-forming stars in the Galaxy. Their work is published on October 29, 2015, in The Astrophysical Journal Letters.
Background information | Questions and Answers | Download links for images |
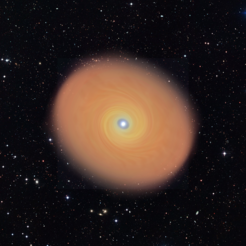
The masses of stars range from close to 10% of the mass of our Sun to more than a hundred solar masses. Do all these stars form in the same way, regardless of their strikingly different sizes? A new discovery by a group of astronomers that includes MPIA researchers has shed new light onto what has been a contentious question for the last few decades.
The team, which is led by Katharine Johnston from the University of Leeds (and formerly a post-doctoral researcher at MPIA) and includes MPIA researchers Thomas Robitaille, Henrik Beuther, Hendrik Linz and Roy van Boekel, found the first clear evidence for a stable disk around a nascent very massive star.
The astronomers observed an object with catalogue number AFGL 4176, a very young, very massive (O type) star in the Southern constellation Centaurus (the Centaur), next to the Southern Cross, at a distance of around 14,000 light-years from Earth.
The inner regions of such very massive young stars are shrouded in gas and dust, but using the ALMA observatory, which observes at submillimeter and millimeter wavelengths, the astronomers were able to take a peek inside, revealing a disk-like, rotating structure. To confirm their discovery, the astronomers created a line-up of more than simulated 10,000 models with different properties. They then compared simulated observations of their "cosmic suspects" with their observational data.
The best fit was a disk containing gas arranged in stable ("Keplerian") orbits around the star (attracted not only by the mass of the star, but also by the inner part of the disk), with a radius of about 2000 times the average Earth-Sun distance, a disk mass of 12 solar masses and a mass of 25 solar masses for the star.
Such disks could play a crucial role in explaining the growth of massive stars - in particular how additional matter can be funneled onto the nascent star in defiance of substantial radiation pressure from the forming star within. Such additional infall of matter is necessary for the formation of the most massive stars known. But up until now, there had be no firm detection of stable disks around the most massive of stellar embryos (that is, the most massive class of young stars: O type stars) to confirm this possibility.
The observations by Johnston and her colleagues send a clear message that at least some of the most massive stars of all can be formed in the same way as all other stars - while there are differences in scales and in timing, the basic mechanisms are the same, and matter is funneled onto the growing young star by a disk.
The high quality of the ALMA observations raises astronomers' hopes for answering some of the remaining open questions concerning the formation of massive stars. In general, detailed observations would allow for a thorough comparison of observations with computer simulations of star formation. More specifically, astronomers hope to catch massive stars in the act of forming twins or companions: almost all very massive stars are part of multiple star systems, with two or more stars in orbit around each other. Future ALMA observations of the kind performed here could be the first to observe tell-tale signs of fragmentation: finding evidence that, as current models predict, stellar multiplets are created in the early stages of star formation.
Background Information
The results described here have been published as Johnston et al., "A Keplerian-like disk around the forming O-type star AFGL 4176" in The Astrophysical Journal Letters.
The co-authors are Katharine G. Johnston (University of Leeds), Thomas P. Robitaille, Henrik Beuther, Hendrik Linz (all Max Planck Institute for Astronomy [MPIA]), Paul Boley (Ural Federal University), Rolf Kuiper (Eberhardt Karls University Tübingen and MPIA), Eric Keto (Harvard-Smithsonian Center for Astrophysics), Melvin G. Hoare (University of Leeds), and Roy van Boekel (MPIA).
Questions and Answers
What is new / important about these results?
Massive stars need effective mechanisms for funneling matter from the surrounding birth cloud onto the surface of the nascent stars - under adverse conditions, since nuclear fusion and the associated radiation pressure set in quite early for this type of star. Accretion disks could be the solution - but so far, only indirect signs have been found that stable disks can even exist around massive stars, and detections so far have been tentative. This is the first firm detection of such a disk around a new-born massive star, and thus a key piece of the puzzle of massive star formation.
The detection does not solve the problem of massive star formation altogether. But it does show that accretion disks are possible around this kind of star. And now that the first such disk has been detected unequivocally, the stage is set for more systematic exploration and statistical analysis, which should then answer the question of how common stable disks are in massive star formation.
What is an accretion disk?
Stars form when the gas in cold interstellar clouds collapses under its own mass. Clouds are never perfectly still; their gas is bound to be in motion, resulting in an overall rotation around the center of mass. As the gas collapses, the rotation speeds up (think of a figure skater going into a pirouette by moving her arms close to her body).
The overall rotation can keep matter from falling onto the would-be star in the same way that planets do not fall straight into the Sun, but instead circle the Sun on regular orbits. But the rotation also creates a mechanism that helps the protostar gather additional mass: the combination of centrifugal forces due to the rotation and the inward collapse flattens part of the gas into a thin disk around the star, known as an accretion disk. This disk can then slowly funnel additional matter onto the would-be star.
The disk is sufficiently dense so as to allow for gas particles within the disk to interact, colliding with each other and transferring some of their energy and momentum in the process. As a consequence of these interactions, there will always be a significant fraction of particles that drift toward the inner rim of the disk and, finally fall onto the protostar in the center. Once the protostar has attracted ("accreted") a sufficient amount of matter in this way, its gas is sufficiently dense and hot for nuclear fusion to set in: a star is born.
What is a stable accretion disk?
In order to play a significant role in the early evolution of the nascent star, the accretion disk needs to be around for a sufficiently long period of time - it needs to be stable, not just a brief, transient phenomenon. Such stable disks settle down into a state that is reminiscent of how planets move in our own solar system: the motion of their gas is dominated by gravity. Consequently, gas in the inner parts of the disk rotates faster than in the outer parts, similar to planetary motion in our own solar system, where the inner planets systematically move faster than the outer ones. Disks that follow this pattern of motion are called Keplerian, after Johannes Kepler, who first formulated the relation between distances and orbital periods for the planets.
For low-mass stars, such accretion disks are well-known, and have been detected numerous times. The most prominent examples are the so-called T Tauri stars, lower-mass nascent stars which have blown away the surrounding gas, allowing for a clear view onto the star and disk. However, it was unclear whether high-mass stars formed in the same way, and therefore were also surrounded by Keplerian disks.
What are the challenges of forming high-mass stars?
High-mass stars are much brighter than their lower-mass kin, and they form and evolve on much shorter time-scales. In fact, in such stars, nuclear fusion sets in after a few hundred thousand years, while there is still plenty of infalling cloud matter - much faster than for their low-mass siblings, which start nuclear fusion (hydrogen burning) much later, only after a few million years.
This early onset of nuclear fusion is a potential problem. In order to form the stars with the highest observed masses, we need to keep adding matter to the newborn star even after nuclear fusion has set in. But nuclear fusion creates intense electromagnetic radiation, and radiation creates pressure (just like, say, a stream of matter particles would - think of turning your garden hose onto a bucket or other object to see this kind of pressure in action). Radiation pressure makes it harder for additional matter to fall onto the star. Sufficiently strong radiation will drive away any remaining matter altogether! Under these adverse conditions, how can stars with very high masses even form?
This is where the presence (or not) of a stable (that is, Keplerian) accretion disk can make a crucial difference. Such disks can funnel additional matter onto the nascent star, after all, and as they are rather thin, they present a much lower profile to the star's radiation pressure than an envelope of gas surrounding the star altogether.
Previously, astronomers observed short-lived, unstable structures around young massive stars. They were toroidal - donut-shaped - resembling a thick ring centered on the protostar, providing high rates of flow that added up to a hundredth of a solar mass per year. While these toroids rotate, they are highly unstable, and their matter falls inward in much less time than it would take for the donut to complete a single rotation. Do these transient structures play a key role for high-mass star formation? Or do massive stars accrete most of their material through stable, Keplerian disks that lie within these toroids? Johnston and her team found one example where this was the case, showing that Keplerian disks can exist around massive forming stars. Now, more state-of-the-art observations are needed with observatories like ALMA, to determine whether this is a common occurrence.
What did astronomers know so far about the possibility of Keplerian disks in young, massive stars?
The answer to the question of whether or not young, massive stars can have stable, Keplerian disks is anything but obvious. In particular, it has long been unknown whether or not the higher radiation pressure and higher rate of matter infall allow for the formation of a reasonably stable (Keplerian) disk in the first place. For some early B-type stars, with masses less than 18 times that of the Sun, disks had indeed been observed since 1997, and their velocity curves, that is how the different parts of the disk move, were clearly Keplerian. But for the most massive stars, O-type stars with more than 18 solar masses, the situation was much less clear.
There have long been indications that disks may be present even around O-type stars. For lower-mass stars, there is a characteristic interplay between the disk and magnetic fields, which results in so-called jets: two highly energetic and focused particle streams that are shot from the innermost parts of the star-disk system in opposite directions perpendicular to the disk. Such jets have also been found for O stars. It is plausible that these jets are created in the same way as for low-mass stars, with the same magneto-hydrodynamic effects in play. Sometimes, more extended outflows have been observed, up to a few light-years long.
Whenever transient rotating structures like the aforementioned toroids are present, the jets are perpendicular to the donut. This relation between the general sense of rotation of the system and jet/outflow orientation is another indication that the mechanism for jet production probably involves rotating disks hidden within the larger toroids.
Taken together, these facts would suggest that nascent O-stars are likely to be surrounded by disks. But a suggestion is not the same as firm evidence.
What is the difficulty with directly detecting Keplerian disks around massive stars, and what instruments are needed for the task?
Direct observations are difficult because of the scales involved. For lower-mass stars, enveloping gas is dispersed before nuclear fusion sets in, leaving only the young star with surrounding disk (a configuration known as a T Tauri star). For higher-mass stars with their more intense radiation and stellar winds, there is only a short time (if any) when the envelope has already been blown away while the disk still remains. If one wants to observe a disk, it will need to be in a state where star and disk are still surrounded by an envelope of gas and dust.
This necessitates observations at longer wavelengths, such as in the submillimeter/millimeter regime. Light at such longer wavelengths can pass almost unhindered through such an envelope, exposing to view the structures that lie within. Such observations not only call for high spatial resolution - that is, access to small details - but also for high sensitivity and high fidelity of the corresponding images. At such comparatively long wavelengths, the high spatial resolution can only be achieved using interferometers: arrays of radio telescopes joined together to act as a single, much larger telescope. Even so, the demands of the disk observations taxed existing interferometers to their limits. This changed with the opening of the ALMA observatory in 2011, producing high sensitivity and excellent quality images in combination with high spatial resolution. Hence, it was anything but a surprise that the past few years produced a number of ALMA-related publications featuring tentative identifications of such disks (Sanchez-Monge et al. 2014, Zapata 2015).
What did the astronomers observe - and why?
The object under study, with the catalogue number AFGL 4176, is an O type star in the Southern constellation Centaurus (the Centaur), next to the Southern Cross. Distance estimates place the object at around 14,000 light-years from Earth. AFGL 4176 is embedded in an extremely bright star formation region, whose total luminosity amounts to approximately 100,000 times that of our Sun. When, in 2012 Katharine Johnston - then a post-doctoral researcher at MPIA - applied for ALMA observation time, she wanted to study the material surrounding the stars in that region. The star-forming region had previously been observed with the MIDI instrument for the VLT interferometer at ESO's Paranal observatory, and ALMA data would complement the existing MIDI data, allowing for comparisons that could reveal different parts of the circumstellar material: While MIDI traces the very inner warm dust, the ALMA observations allow for the study the colder dust and gas. In particular the latter are crucial to investigate the kinematics of the gas around the forming star.
In addition to tracing interstellar material, the astronomers had also hoped that they might find a disk structure - and then find out whether that disk was affected by the presence of hot ionized gas in the surrounding region. The observations targeted certain frequencies of light (spectral lines) characteristic for methyl cyanide (an organic solvent that is also known as acetonitrile, chemical formula CH3CN). Light at these frequencies is only emitted from regions that are dense and have temperatures between tens and hundreds of Kelvins; it can thus be used to trace denser structures within gas clouds. In the ALMA observations, most of the dense gas detected in this way was at the same location as a prominent infrared source. This was a tantalizing hint that there might indeed be a disk of gas orbiting a protostar (which corresponds to the infrared source). But to find out whether this was indeed a rotating disk, additional analysis of a different kind was needed.
ALMA observations usually include spectral information, that is, information about the distribution of energy among the various frequencies of the observed radiation. But motion leads to systematic shifts in light frequency: due to the Doppler effect, we see light from a source that is moving toward us at somewhat higher frequencies (blueshift), light from a source that is moving away at lower frequencies (redshift). For the characteristic light due to methyl cyanide, the wavelength for a source at rest can be determined in the laboratory; therefore when astronomical observations show this spectral feature shifted to lower or higher wavelengths than that rest frequency, the gas emitting the light is moving either towards or away from the observer.
Using the ALMA observations, the astronomers were able to create velocity maps, in effect a kind of Doppler shift map where for each image pixel, the average radial motion of the light-emitting gas in that region is tracked. For AFGL 4176, this kind of map showed the characteristic signature of a disk seen somewhat from the side (inclination angle 30 degrees), with one half of the disk rotating towards us, the other half away from us.
How did the astronomers analyze their data to show the presence of a stable, Keplerian disk?
To show that their find was indeed a Keplerian disk, with a sharp increase of rotational velocity in the disk's inner regions, Johnston and her colleagues began to create models for the disk, with different disk masses, disk radii, distributions of mass within the disk, viewing angles and envelopes of gas around the disk. These models incorporate the physics of what is happening in the disk: the spectral type of the star determines the amount of radiation emitted; the radiation in turn heats up the disk, determining disk temperature; the disk shape is a consequence of pressure (which follows from temperature) and the gravitational attraction of the star.
In this way, the researchers obtained more than 10,000 individual models (1512 models, seen from 7 different angles), covering a variety of different possible situations: disks seen from angles of 10, 20, 30, 40, 50, 60 or 70 degrees; a range of more massive and less massive disks, with different kinds of additional envelopes of surrounding gas, and many more variations besides.
To simulate the appearance of each such disk, the astronomers used radiative transfer models, which simulate how radiation moves through a given configuration of gas and dust, being absorbed and re-emitted, and heating up the various parts of matter (thus creating additional thermal radiation) in proportion to the energy received in each region. As a last step, the astronomers needed to simulate the ALMA observations, arriving at a faithful simulation of how each of those 1512 hypothetical disks would look when observed with ALMA: a simulated ALMA image plus simulated measurements showing spectral lines of varying positions, widths and brightnesses.
The final step was to match simulated with actual observations. The model which produces the best observational match, the reasoning goes, is likely to be the best match for the physical properties of the disk, e.g. mass, radius, viewing angle, and properties of the surrounding gas. The best fit corresponds to models that are close to the following values: a disk in Keplerian orbit around the star (attracted not only by the mass of the star, but also by the inner part of the disk), with a radius of about 2000 AU (that is, 2000 times the average Earth-Sun distance) and a total disk mass of 12 solar masses, its surface density decreasing in a specific way with the radius (1/r1.5). The star itself was found to have a mass of around 25 solar masses.
What are possible directions for future research?
The quality of the ALMA observation sets the scene for the next stage, which would involve a direct comparison between simulations of how stars form over time (which typically involve equations linking gravity, hydrodynamics, and magnetic fields within the disk) and observations of young massive stars and their disk - discrepancies would point towards areas where our current understanding of the formation of massive stars would be incomplete or flawed.
The superb resolution of ALMA, allowing for the mapping of the innermost regions of the nascent star system in great detail, is certainly needed for another key observation concerning massive stars: 96% of all O stars are part of binary systems or even larger multiple star systems. Just as human twins or multiplets form in the womb, fragmentation leading to the formation of multiple stars is thought to occur while matter is still accreting onto the protostar. Higher-resolution observations of the disks of massive protostars could show structures - such as spiral arms – that are conducive to fragmentation, or even the fragmentation into several cores itself that would indicate the formation of companion stars (or instead massive planetary companions of a massive star). Such direct observation of fragmentation would be the next big observational advance in understanding the formation of massive stars.
Download area
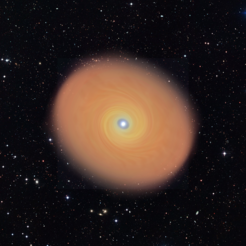
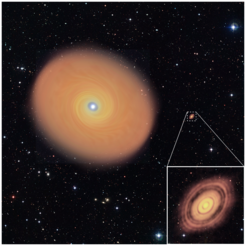