How Planets Can Form Quickly Despite Difficult Circumstances
Scientists of the Max Planck Institute for Astronomy in Heidelberg have found a surprising solution for a fundamental problem in the formation of planets, working together with colleagues from the USA and Canada. The results appear in the 30 August 2007 issue of Nature(1).
Planets are built up from tiny dust grains in gas disks around young stars. This growth process is a mystery in modern astrophysics because it was not understood how to get from meter-sized boulders to kilometer-sized »planetesimals«. In order to solve this puzzle, the Heidelberg researchers and their colleagues used a supercomputer to model the physical processes occurring in disks around young stars. They took into account all the forces acting on the gas and dust, as well as the interactions between them, in a computationally intensive, three-dimensional model of the growth process. In contradiction to previous assumptions about the nature of turbulence in the disks the researchers found the surprising result that the boulders were concentrated in the turbulent flow. These overdense regions collapsed due to the combined gravity between the boulders, to directly form thousand-kilometer-sized dwarf planets.
Planet formation is a side effect of star formation. A large cloud of interstellar gas and dust becomes unstable and begins to collapse towards its center of mass under its own weight. The material originally dispersed across a vast volume of space has some angular momentum (it rotates around a specific axis running through the center of mass). As a result, the gas and dust collapses fastest along the rotation axis, while perpendicular to it, in the equatorial plane, it is delayed by centrifugal force, which becomes stronger as the material falls further in. The result is the formation of a new star in the center from most of the collapsing material, while a thick disk of gas and dust remains in the equatorial plane, rotating around the central star. The rotation speed of the disk is highest on the inside, and falls off with increasing distance from the central star. Such disks can be seen with modern telescopes, and are already being studied quite carefully.
The dust particles embedded in the dense disk rotating around the central star frequently collide with each other, and stick together. They grow (through »adhesion«) to larger and larger objects. This form of growth ends, however, as soon as meter-sized chunks appear in the disk. Such boulders hardly ever stick together when they collide; they even destroy each other instead. Further, because the surrounding gas impedes their orbital motion around the central star, they follow spiral orbits that lead them to fall into the central star within a few centuries.
Until recently researchers saw no way this »meter-size barrier« could be crossed. The theory of planet formation ended in a blind alley. Only after the formation of planetary embryos from the collisions of kilometer-sized »planetesimals« is the story of the planets again understood.
However, in nature this barrier between meter-sized boulders and many kilometer-sized planetesimals clearly must be crossed – the proof lies in the existence of the eight planets of our Solar System (and the 200 planets around other stars that have been discovered).
In order to solve the puzzle, the Heidelberg researchers and their colleagues used a supercomputer to model the physical processes occurring in the circumstellar disk. They took into account all the forces acting on the gas and dust, as well as the interactions between them, in a computationally intensive, three-dimensional model of the motion of a huge number of individual boulders.
Magnetic fields already thread the system before the beginning of the collapse, and are strengthened by the collapse. These fields drive turbulence in the flows occurring within the disk. The turbulence in turn produces regions in which the gas pressure is slightly higher than the surroundings. These pressure variations act to change the speed of the boulders, leading to clumping in the boulder layer, just as a narrowed lane on a freeway easily produces a traffic jam. The clumps in the boulder layer couple with their collective velocity back to the gas velocity, which leads to a further concentration of the boulders.
So many boulders come together in these local condensations that their gravitational attraction to each other overwhelms all other effects. The boulders form bound clusters with the mass of asteroids or dwarf planets. These are massive enough that the gas can no longer brake them. The growth process is astonishingly effective, finishing in just approximately a hundred years.
It can be expected that the boulder clusters quickly condense into solid bodies. These bodies are the »planetesimals« which at one time populated the entire disk. Successive collisions among the planetesimals create the first planetary embryos. Some of the embryos grow large enough to attract gas and the remaining planetesimals by their gravity and grow to full-fledged planets. Comets and asteroids are believed to be the last survivors of the ancient planetesimal cloud.
The researchers quantitatively followed the passage of objects through the meter barrier by turbulence and local condensation using their complex and detailed computer simulations. This does not yet prove that nature actually follows the scenario summarized here. That can only be decided by future observations. However, this work does provide for the first time a realistic picture of a possible road to planet formation in circumstellar disks of gas and dust.
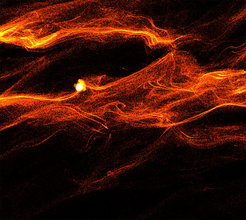
- Anders Johansen(2), Jeffrey S. Oishi(3,4), Mordecai-Mark Mac Low(3,2), Hubert Klahr(2), Thomas Henning(2), and Andrew Youdin(5): Rapid planetesimal formation in turbulent circumstellar discs. Nature, MS Nr. 2006-12-13825D.
To be published in Nature, August 30, 2007. - Max Planck Institute for Astronomy, Heidelberg, Germany
- American Museum of Natural History, Department of Astrophysics, New York, USA
- Also Department of Astronomy, University of Virginia, Charlottesville, USA
- Canadian Institute for Theoretical Astrophysics, University of Toronto, Canada