Numerical simulations of planetesimal formation reproduce key properties of asteroids, comets
With simulations that go into finer details than ever before, Brooke Polak of the University of Heidelberg and Hubert Klahr at the Max Planck Institute for Astronomy (MPIA) have modeled a key phase in the formation of planets in our solar system: the way that centimeter-size pebbles aggregate into so-called planetesimals tens to hundreds kilometers in size. The simulation reproduces the initial size distribution of planetesimals, which can be checked against observations of present-day asteroids. It also predicts the prevalence of close binary planetesimals in our solar system.
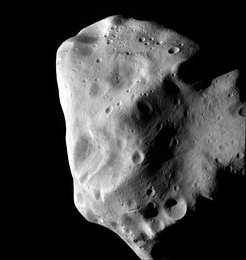
In a new study published in the Astrophysical Journal, astrophysicists Brooke Polak from the University of Heidelberg and Hubert Klahr from the Max Planck Institute for Astronomy used simulations to derive key properties of so-called planetesimals – the intermediate-size bodies from which planets formed in our solar system roughly 4.5 billion years ago. Using an innovative method for simulating planetesimal formation, the two researchers were able to predict the initial size distribution of planetesimals in our solar system: how many are likely to have formed in the different “size brackets” between roughly 10 km and 200 km.
Several groups of objects in today's solar system, specifically the main-belt asteroids and the Kuiper Belt objects, are direct descendants of planetesimals that did not go on to form planets. Using existing reconstructions of the initial size distribution of the main-belt asteroids, Polak and Klahr were able to confirm that their prediction indeed matched observations. In addition, their model makes successful predictions for the differences between planetesimals formed closer to the Sun vs. those formed farther away, as well as predicting how many form as binary planetesimals.
Planet formation from dust to planets
Planet formation around a star proceeds in several stages. In the initial phase, cosmic dust particles in the swirling protoplanetary disk around a new star clump together, bound by electrostatic (van der Waals) forces, to form so-called pebbles a few centimeters in size. In the next phase, pebbles join together to form planetesimals: space rocks between tens and hundreds of km in diameter.
For these larger objects, gravity is so strong that collisions among individual planetesimals form even larger, gravitationally-bound, solid cosmic objects: planetary embryos. These embryos can continue to accrete planetesimals and pebbles until they become terrestrial planets like Earth. Some may go on to accrete thick layers of mostly hydrogen gas to become so-called gas giants like Jupiter, or ice giants like Uranus.
When planetesimals do not become planets
Not all planetesimals become planets. One phase of solar system history involved the newly-forming Jupiter, today the solar system's largest planet, migrating inward, towards a closer orbit around the sun. This migration disrupted planet formation in its direct vicinity, with Jupiter's gravity preventing nearby planetesimals to evolve into planetary embryos. Uranus and Neptune also migrated, but outwards to more distant orbits, as they interacted with the planetesimals beyond them. In the process, they scattered some of the more distant, icy planetesimals into the interior solar system, and some outwards. Quite generally, far from the Sun, typical distances between planetesimals were too far for even the relatively small Earth-like planets to form – the only planetary embryos that formed were even smaller objects like Pluto. Most planetesimals at that distance did not make it to the planetary-embryo stage at all.
In the end, our solar system ended up with several regions containing left-over planetesimals or their descendants: the main asteroid belt between Mars and Jupiter contains both planetesimals that Jupiter kept from forming embryos and those scattered inward by Uranus and Neptune. The disk-like structure of the Kuiper belt, between 30 and 50 astronomical units (=Earth-Sun-distances) from the Sun contains planetesimals too far out to be disturbed by the migrations of Uranus and Neptune, approximately 70.000 of them with sizes larger than 100 km. This is where most medium-period comets that visit the inner solar system come from. Further out, in the so-called Oort cloud, are objects that were scattered outwards by the Uranus-Neptune migration.
The limitations of planet-formation simulations
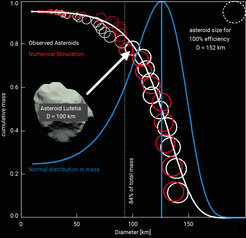
Simulating the progression from centimeter-size pebbles to planetesimals is challenging. Until about a decade ago, it wasn't clear how that transition could happen in the first place – back then, simulations did not allow pebbles to grow beyond a size of about one meter. That particular problem has since been solved, with the realization that turbulent motion in the protoplanetary disk brings a sufficient amount of pebbles together to form larger objects. But the disparate scales involved still make simulations of planet formation very difficult.
Continuum simulations model the protoplanetary disk by dividing space into a grid of separate regions – the three-dimensional analogue of dividing a plane into a chessboard pattern. One then uses the equations of hydrodynamics to compute how matter flows from each grid cell to neighboring cells, and how matter properties change during that process. But in order to obtain meaningful results, one needs to simulate a section of the protoplanetary disk hundreds of thousands of kilometers in diameter. There is simply not enough computing power to make the “chessboard pattern” sufficiently small for simulating the kilometer-scale structure of individual planetesimals at the same time.
One alternative are simulations that model groups of pebbles as separate "super particles," and then merge them into single point-like objects once they approach each other closer than a limit of about 1000 km. But this method fails to capture another important aspect of planetesimal formation: close binary planetesimals, where two planetesimals orbit each other closely or even come together as "contact binaries."
Simulating a “pebble gas”
The simulations undertaken by Polak and Klahr go in a different direction, borrowing concepts from a seemingly unrelated physical model: the kinetic description of a gas, where myriads of molecules fly around at high speeds, their collisions with the sides of a container cumulatively exerting pressure on the container walls. When the gas temperature is low enough and the pressure high enough, a gas undergoes a so-called phase transition, becoming liquid. Under certain conditions, the phase transition can take a substance directly from the gaseous to the solid state.
Polak's and Klahr's simulation treated small groups of pebbles in a collapsing cloud in a protoplanetary disk analogously to particles of this kind of gas. Instead of modeling the collisions between the various pebble groups explicitly, they assigned a pressure to their "pebble gas." For the so-called equation of state, which gives the pressure as a function of the density, they chose a so--called adiabatic equation of state – the kind of equation that, in a spherically-symmetric situation, has a density structure similar to that of Earth’s. With this choice, the pebble gas can undergo a phase change as well: At low density, there is a "gas phase" in which separate pebbles fly around and collide frequently. Increase the density, and you can make the transition to a "solid phase," where the pebbles have formed solid planetesimals. The key criterion for when the pebble gas becomes solid is whether or not the gravitational attraction of the pebbles is greater than the pressure sustained by the collisions.
Planetesimal properties depend on the distance from the Sun
Earlier work in Hubert Klahr's group had shown that planetesimal formation always begins with a compact cloud of pebbles within the protoplanetary disk collapsing in on itself – and also yielded concrete values for the sizes of such separate collapsing regions. In this new work, Polak and Klahr look at several versions of such a collapsing region, each with at a different distance from the Sun, starting with a distance as close as Mercury's orbit and ending with a collapsing region as far away as Neptune.
As their simplified equations are much less complex than those of super-particle collisional models, the researchers were able to use their available computing power to simulate finer details than ever before – right down to the scales on which binary planetesimals can form as contact binaries. Previous simulations, lacking the capacity of tracking down such fine details, would just assume that two planetesimals getting as close as is necessary to form a close binary would have morphed into a single structureless object, and thus would miss those close binaries altogether.
Predicting the size distribution of planetesimals
Their results paint an interesting picture of planetesimal formation as a whole. Distance from the Sun is key: a collapsing region very close to the Sun will produce only a single planetesimal. At greater distances, each collapsing region will form more and more planetesimals at the same time. Furthermore, the largest planetesimals form closest to the Sun. The largest planetesimals produced by a collapsing pebble cloud at the Earth's distance from the Sun are around 30% more massive and 10% larger than those produced ten times farther out. Overall, planetesimal production turns out to be very efficient, with more than 90% of the available pebbles ending up in the resulting planetesimals regardless of location in the solar system.
The simulation's prediction for the size distribution of planetesimals is spot-on. Of course, even for the main belt asteroids, life went on over the past billion years, with numerous collisions busting larger planetesimals into smaller fragments. But analyses that aim to reconstruct the initial size distribution from what is seen today come to very similar results as the new simulations.
And there was one surprise: "Previously it was thought that the initial size distribution among the asteroids reflects the mass distribution of the pebble clouds," says Brooke Polak, "so we were very surprised that our simulations, always using the same initial mass for the pebble clouds, created the same mass distribution of asteroids during the gravitational collapse in as is found in observations. This dramatically changes the constraints on the processes that create the pebble clouds in the solar nebula." In other words: simulations of the earliest stages of our solar system will not need to worry about getting the pebble cloud sizes just so – planetesimal formation will take care of the proper size distribution on its own.
Binaries and moons
The eye for detail that Polak's and Klahr's simulation has built in has also yielded unprecedented results about binary planetesimals, with pairs of planetesimals orbiting each other. Half of the binaries are very close to each other, their mutual distance less than four times the diameter of the planetesimals themselves. Predictions for the prevalence and properties of binaries, including binaries with additional small "moons" orbiting them, neatly match the observed properties of Kuiper-belt objects in the outer reaches of the solar system, as well as those of main-belt asteroids.
One of the predictions is that close binaries form in great numbers early on, as the pebbles coalesce to planetesimals – as opposed to forming through later near-collisions and other interactions. The NASA space mission Lucy, which was launched in 2021, promises a particularly interesting opportunity of testing this prediction. "Not all planetesimals end in the Asteroid or Kuiper Belt. Some get trapped in a co-orbit with Jupiter itself, the so-called Trojans." says Hubert Klahr. "The Lucy mission will visit several of them over the next years. In March 2033, it will swing by the asteroids Patroclus and Menoetius. Each is 100 km in size, and the two orbit each other at a distance of only 680 km. Our prediction is that these two will have the same colour and outer appearance, as we expect that they formed from one and the same pebble cloud. Identical twins since birth."
Future directions for research
The present version of the simulations of Polak and Klahr only examines planetesimal formation out to about the present orbit of Neptune. Next, the two researchers plan to explore the early history of our solar system at even greater distances. While the present simulations already yield objects like the contact binary Arrokoth, which was visited by NASA’s New Horizons probe in 2019 after its visit to the Pluto-Charon system, it would be interesting to see how objects like this could form at Arrokoth’s actual orbital distance – 45 times as far from the Sun as Earth (as opposed to Neptune’s 30 times).
Another limitation of the present simulation is that planetesimals can only form as perfect spheres of different sizes. A more sophisticated equation of state that incorporates the ability of solid bodies to keep their shape would allow for a description of objects with the material properties of a mix of porous ice and dust. On this basis, the calculations could be extended to planetesimals of varied shapes, allowing even more details between our understanding of solar system formation and observations.
Background information
The results described here have been published as B. Polak and H. Klahr, “High Resolution Study of Planetesimal Formation by Gravitational Collapse of Pebble Clouds” in the Astrophysical Journal.
Hubert Klahr leads the Planet and Star Formation Theory group at MPIA, while Brooke Polak is a PhD student of the International Max Planck Research School (IMPRS) Heidelberg at the Institute for Theoretical Astrophysics at Heidelberg University, and currently a guest scientist at the American Museum of Natural History in New York.