Ultracool disk around young star contains dusty surprises
While the circumstellar disk in question has been nicknamed "the Flying Saucer," what is most mysterious about it are not extraterrestrials, but tiny particles of cosmic dust. An unusual new measurement of the disk's dust temperature using the ALMA observatory has yielded surprisingly low values, a mere 7 degrees above absolute zero (7 K). The astronomers, including Dmitry Semenov of the Max Planck Institute for Astronomy, found that the only viable explanations involve unusual properties of the disk's dust grains. With these unusual properties, dust disks could be quite generally more massive than previously thought, with consequences for the types of planets that can be born in such disks.
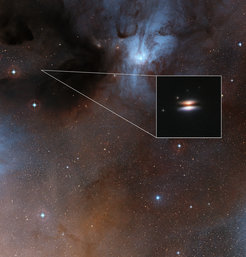
The Rho Ophiuchus star formation region at a distance of about 400 light-years from Earth. The inset shows an infrared image of the Flying Saucer protoplanetary disk, taken with the Hubble Space Telescope. Recent observations of this disk have revealed dust in that disk to have an unexpectedly low temperature.
The protoplanetary disk nicknamed the Flying Saucer not only looks unusual on astronomical images. When astronomers observed it with the ALMA observatory, it seemed to have a highly unusual property: at face value, the measurements showed that the astronomers were receiving a negative amount of radiation from the object. While that absurdity was readily explained by peculiarities of the ALMA measuring process, there is a serious background to the negative values: They show that, contrary to expectations, dust in this disk is colder than the molecular clouds in the background.
This is highly unusual in and of itself. After all, the disk is constantly warmed by the star in its center. For its dust to be this cold, a mere 7 degrees Celsius above absolute zero (= 7 Kelvin), the dust grains must have some unexpected properties.
When Stephane Guilloteau of the University of Bordeaux and Thomas Henning of the Max Planck Institute for Astronomy had first proposed these observations, they had indeed intended to measure dust temperatures in the disk. But they had never expected temperatures this low.
Dmitry Semenov of the Max Planck Institute for Astronomy, one of the study's authors, explains: "The usual models assume that dust grains are compact and spherical. But a disk made of such grains, around a Sun-like star, could never have temperatures that low. Hence, the low temperatures that have been measured point towards a more complex situation. The dust grains could be compact but non-spherical - for instance: they could be elongated, or be highly porous aggregates made of small compact grains. Alternatively, the central regions of the Flying Saucer could contain unusually large dust grains that are thermally decoupled from the surrounding gas, and remain very cold."
At present, there is not enough data to decide between those possible explanations. Further observations are planned – not least because of the ramifications: Protoplanetary disks are the birthplaces of planets, after all. Dust properties play a key role in the first steps of planet formation, where grains of dust clump together to form slightly larger compounds, setting off the sequence that ends with the formation of large planets. The surfaces of dust grains are miniature laboratories, the site of complex chemical reactions including those that form organic compounds, which in turn could prove crucial for the formation of life on one of the system's planets.
Last but not least, a widespread method of estimating the total mass of such disks, most of which is in the form of molecular gas, relies on detecting radiation from the dust, estimating the amount of dust present, and using a conversion factor to estimate the gas mass. This conversion, too, might need to be modified when the dust turns out to have unusual properties – altering estimates of disk mass and thus of what planets that can form in a particular disk.
All good reasons to know your dust if you're interested in planet formation – and when it comes to that, the recent Flying Saucer measurements provide a wake-up call, showing that dust could well be less simple than commonly assumed.
Background information
The results described here have been published as S. Guilloteau et al., "The shadow of the Flying Saucer: A very low temperature for large dust grains" in Astronomy & Astrophysics Letters.
An in-depth description of the results can be found here.
The MPIA researchers involved are
Dmitry Semenov, Thomas Henning, and Til Birnstiel
in collaboration with
S. Guilloteau (Université de Bordeaux/CNRS, Floirac), V. Piétu (IRAM, Saint Martin d’Hères), E. Chapillon (Université de Bordeaux/CNRS; IRAM), E. Di and A. Dutrey (beide University of Bordeaux/CNRS), and N. Grosso (Observatoire Astronomique de Strasbourg).
In-depth information: Ultracool disk around young star contains dusty surprises
Going back to basics, astronomers measure the radiation their telescopes receive from different directions in the sky. With this setup, one would expect each measurement to yield a positive or zero value. Either radiation is reaching us from a certain part of the sky or it isn't.
An unnatural negative value and its explanation
That is why, at first glance, measuring a negative value for the radiation received from a certain celestial object sounds absurd. But that is what happened when a team of astronomers led by Stephane Guilloteau (Laboratoire d'Astrophysique de Bordeaux, France), which includes Dmitry Semenov, Thomas Henning and Til Birnstiel of the Max Planck Institute for Astronomy, used the ALMA observatory in Chile to observe the protoplanetary disk nicknamed "The Flying Saucer," which surrounds the young star 2MASS J16281370-2431391 in front of the dark nebula known as the Rho Ophiuchi cloud complex. The cloud complex is located in the constellation Ophiuchus near the celestial equator, and located at a distance of about 400 light-years from Earth. Protoplanetary disks are the birthplaces of planets: disks of gas and dust surrounding young stars; inside these disks, smaller particles clump to form larger objects, eventually reaching planetary size over the course of some millions of years.
What, then, about the negative value? Part of the solution lies in the special properties of ALMA, which uses the technique known as interferometry to link 66 separate radio dishes deployed on the Chajnantor plane in the Chilean Andes, nearly 5000 meters above sea level. The short version is that by linking these dishes, which are built to receive submillimeter and millimeter radiation, ALMA can act as a telescope with unparalleled high resolution for cosmic detailed. Usually, the fine details visible to a telescope are limited by the telescope's size. In interferometry, the limit is set by the maximum separation of the single dishes that have been linked together. In its widest configuration, ALMA can see the same amount of detail accessible to a single radio dish 16 kilometers across!
Rarely mentioned, but crucial in this particular case, is that the gain in fine details comes at a price. Depending on the placement of various antennas, it is possible that ALMA will be able to detect small details, but will be largely blind to more gradual changes. In the case of the Flying Saucer observations, ALMA was able to pick out minute details, but was largely blind to the fact that there was a fairly constant brightness throughout its whole field of view, caused by one of the Rho Ophiuchi molecular clouds which lies directly behind the Flying Saucer disk.
An unusually cold disk
The negative measurement values, then, are no more than the fact that the disk (which ALMA can see) actually gives off less radiation than the cloud in the background (which ALMA cannot see). While this solves the most fundamental problem, the fact that the protoplanetary disk gives off less radiation is surprising in and of itself. Molecular clouds are typically very cold, namely in the range of 10 to 15 Kelvin, which amounts to -258 to -263 degrees Celsius, 10 to 15 degrees Celsius above absolute zero
The negative measurement values indicate that the Flying Saucer dust disk must be colder still, at 5 to 8 Kelvin. Going by most models of such disks, this is unexpectedly low. There is, after all, a star at the center of the disk, irradiating the disk with its light. This will inevitably warm up the disk, with the amount of warming depending on the disk's properties. The process is analogous to the way that everyday objects left out in the Sun will get warmer, some more, some less.
Astronomers can calculate the degree of disk warming using what is known as radiative transfer models. These are certain types of simulations which trace the effects of the star's radiation through the disk, keeping track of scattered and absorbed light, of the warming-up of different disk regions, and of thermal radiation associated with the temperature of different parts of the disk.
Compared with the results of such models, dust temperatures in the Flying Saucer disk are very low indeed. In the simplest models, which assume small, spherical dust grains, they are downright impossible.
A case of more complex dust
At this point, the astronomers turned to Dmitry Semenov, an astrochemist and astrophysicist at the Max Planck Institute for Astronomy. Semenov is an expert in the chemical processes taking place in such dust disks, in which dust grains and their surface properties play an important role.
Semenov was able to tell them that indeed, in modern physics-based models, dust temperatures in disks could not drop that low unless there was some way for the dust to emit electromagnetic radiation more efficiently than usual at longer wavelengths. One solution is contained in work that Semenov did back in 2000, when he was a physics student at St. Petersburg University, studying the interaction of radiation with the dust grains found in the tails of comets.
Most models assume dust grains to be spherical and compact. But Semenov, in his work on comets, studied more complex dust grains, as well. His results could serve to explain the low temperature of dust in the Flying Saucer disk: for dust grains made of electrically conducting material (such as certain organic compounds, or compounds containing iron), either non-spherical, elongated dust grains or else highly porous grains (think of Swiss cheese!), possibly aggregates of smaller compact grains, would be able to radiate more efficiently at longer wavelengths, cooling down the dust. Even near the midplane of the disk, where dust is buffeted around by the surrounding gas, this could lead to very cold dust grains (that would be colder even than the surrounding gas!).
Semenov's is not the only possibility for the ultra-cool Flying Saucer. Alternatively, it could be that there is a temperature difference between larger dust grains on the one hand and smaller grains on the other. Collisions with the gas of the disk would not be sufficient to bring the two kinds of grains into thermal equilibrium within the time scales involved.
With the data at hand, it is too early to decide which of the alternatives is the valid explanation. We should learn more from additional observations with ALMA and other observatories that are currently in the planning stage.
Dust with consequences
A lower-than-expected dust temperature might not sound like a big thing, but it could have significant consequences for our understanding of how planets form.
One reason is that astronomers cannot directly measure the amount of gas contained in a protoplanetary disk such as the Flying Saucer. One common estimate amounts to measuring the mass of dust contained in the disk, going by the thermal radiation emitted by the dust, and then extrapolating to the gas mass using a heuristic conversion factor. But changed dust properties could mean that this simple conversion is flawed at least in some of the cases to which it is commonly applied. This in turn could greatly influence the mass estimates for protoplanetary disks, which in turn are important for predicting which kinds of planets (massive or less massive? closer to the star or farther away?) are likely to form.
Furthermore, grain properties are the key to the earliest stages of planet formation. These stages involve dust grains clinging together, forming slightly larger conglomerates. Clumping behavior will depend on the shape and surface properties of the grains, including the presence of water ice, which is thought to serve as a kind of glue at certain stages of the clumping process.
Another interesting consequence concerns disk chemistry. Dust grains, with their comparatively large surfaces, are thought to be important mini-laboratories in the disk, ideally suited for the formation of molecules, including more complex organic molecules. It is even possible that dust chemistry is responsible for creating some of the chemical compounds that will later on be crucial for the formation of life on a putative planet that has formed from the disk.
Changing the dust properties would change the laboratory in which these compounds are formed. For instance, on cold dust grains, surface reactions will not proceed as efficiently as on warmer grains, and some organic reactions would become so slow as to become insignificant over the time scales involved. If this is the case, the organic molecules that astronomers find in comets and in carbon-rich (carbonaceous) meteorites would have needed to form another way than on dust grains in the protoplanetary disks – such as inside carbon-rich asteroids in the presence of liquid water. But whatever the details, the message is clear: whoever wants to understand chemistry in such protoplanetary disks had better know the shapes, surface properties, and temperatures of the dust grains involved!