Probing the three-dimensional structure of a protoplanetary disk
Astronomers have detected three-dimensional structures in the protoplanetary disk around the young star TW Hydrae. Their pioneering reconstruction uses both the light reflected by dust and light emitted by certain gas molecules to reconstruct the disk’s physical structure – a step forward from previous images of such disks that could not distinguish density variations from chemical or thermodynamic patterns. The pattern, a ring-shaped gap, could either indicate the presence of a planet or instabilities that could form a planet.
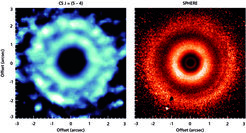
Planets form in swirling disks of gas and dust – and the spatial structure of such a disk holds valuable clues to the mechanisms of planet formation. It is only over the last few years that astronomers have gained the ability to take images that are detailed enough to show not only the disks, but some of their structure.
Now, astronomers led by Richard Teague, a graduate student at the Max Planck Institute for Astronomy, have taken the next step. Previous images of disk structures, impressive as they were, could not reliably distinguish whether certain disk regions looked different because of different matter properties (e.g. larger or smaller dust grains) or whether the different appearance was due to a density variation.
Density variations are particularly interesting because they could indicate the presence of a young planet, or of an area where a new planet is likely to form. Teague and his colleagues combined different kinds of light, namely light scattered by dust particles and light emitted by carbon monosulfide molecules, to demonstrate the presence of a ring-shaped gap where the density is less than half of the adjacent disk regions.
The gap is far out: about 95 times as far away from the central star as the Earth is from the Sun. Both the presence of a planet and the occurrence of planet-forming processes this far out pose a challenge to current models of planet formation, which do not account for planets forming that far out in this kind of disk.
The work by Teague and his colleagues and a similar work, published in December 2016 by Andrea Isella and team at Rice University in Texas, open a new era of study of planetary birthplaces, in which astronomers begin to probe the three-dimensional physical structure of these disks.
Background information
The results described here have been published as R. Teague et al.: "A Surface Density Perturbation in the TW Hydrae Disk at 95 au Traced by Molecular Emission" in Astrophysical Journal 835 (2017), 228.
The research was carried out by Richard Teague, Dmitry Semenov, Thomas Henning, Tillman Birnstiel, Roy van Boekel (all MPIA) in collaboration with Uma Gorti (NASA Ames and SETI-Institut), Stéphane Guilloteau, Anne Dutrey (both University of Bordeaux and CNRS), and Edwige Chapillon (University of Bordeaux, CNRS, and IRAM).
In-depth description: Probing the three-dimensional structure of a protoplanetary disk
Planet formation
Planets form in protoplanetary disks: gigantic disks of gas and dust surrounding young stars. In these general terms, astronomy's basic scenario for planet formation has been around since the 19th century. But since then, our understanding of just what these protoplanetary disks are, and how planets form inside them, has made great strides – many of them linked to progress in observational techniques. The latest advance is just a few years old: Recently, images of protoplanetary disks have become sharp enough to show us details of the structure of such disks.
In the research on protoplanetary disks, the disk around the star TW Hydrae has long played a special role. Akin to the Rosetta Stone in Egyptology and Drosophila fruit flies in genetics, the TW Hydrae disk is a particularly readily accessible and, in consequence, well studied specimen of a protoplanetary disk. It is just 190 light years from Earth (and thus 2.5 times closer than the next nearest disk), surrounding a young star with about the same mass as the Sun; a chance to study planet formation at comparatively close range. In consequence, TW Hydrae has one of the most frequently observed protoplanetary disks of all, and these observations play an important role as a touchstone for current models of planet formation.
Images showing substructure...
Since 2013, astronomers have been able to obtain images showing various aspects of the spatial structure of the TW Hydrae disk. Particularly interesting are recent images that show ring structures, including gaps, and localized lumps (cf. this MPIA press release from March 2016); in other disks, astronomers have even found spiral structures reminiscent of spiral galaxies (cf. this MPIA press release from September 2016).
These structures could represent disk regions with more than average matter density and with less than average density, and that would link them directly to the formation of planets, for two reasons: Very likely, local regions of greater-than-average density are needed to explain the formation of planets in the first place. Secondly, once a planet has grown to a certain mass, its gravity will have observable effects on the structure of the disk, which could manifest as rings, gaps, or lumps.
...or is it just chemistry and dust properties?
However, when it comes to images of protoplanetary disks, what you see is not necessarily what you get. A ring, for instance, could also mark a snowline: Inside the ring, it is warm enough for a certain species of molecule to exist as a gas; outside, the molecules are in a frozen state, clinging to the disk's dust particles.
The evolution of the dust particles themselves provides another possible mechanism: As dust particles clump together, their size changes. A local difference in particle size can change the amount of light emitted or reflected by that region of the disk. The resulting local structure would be visible in observations, but would not necessarily represent a density variation.
Until recently, disk structures were identified with one kind of observation only – mostly the detection of light reflected by the dust that makes up about 1% of matter in the disk. That is insufficient information to exclude alternative (chemical, thermodynamical) explanations for the observed structures.
Targeting the outer regions of the TW Hydrae disk
Now, a study led by Richard Teague (MPIA) has targeted the outer regions of the disk around TW Hydrae in a way that drastically reduces the ambiguities and allows the astronomers to identify three-dimensional disk structures, notably a gap with markedly reduced density, with considerable certainty.
The astronomers' initial goal had been something else entirely. In current models of planet formation, turbulence plays a key role, creating small-scale inhomogeneities that can serve as the seeds of planet formation. Teague and his colleagues set out to measure the turbulence within the TW Hydrae disk.
To this end, the researchers had observed light, specifically: spectral emission lines coming from the molecules carbon monoxide (CO) an carbon monosulfide (CS) as well as from cyano radicals (CN), using the ALMA observatory. Located in the Atacama desert in Chile, ALMA is an array of more than 60 radio telescopes tied together to work as a single, large telescope.
Scratching the surface – and below
Why these specific types of light, more precisely, these spectral lines? Because, depending on the properties and the abundances of certain molecules, the specific light emitted by these molecules conveys information about the three-dimensional structure of the disk.
CO has a comparatively high abundance. That means there are plenty of CO molecules around to absorb and scatter light from spectral lines that are specific to CO. This makes the disk opaque for its specific light: Using the CO lines, observations will only show light from CO at or near the disk surface. On the other hand, for some of the lines, notably CS, the whole disk is virtually transparent, and observations will show light from all the CS molecules in the disk. The CN lines are somewhere in between. For them, the disk is semi-opaque, and these lines will convey information down to some depth beneath the disk surface, but not about the whole of the disk.
By combining observations in all three lines, one can obtain at least some information about the differences between the surface, deeper layers, and the disk as a whole – in short: information about the three-dimensional structure. Using this information, the astronomers concluded that the disk indeed showed signs of turbulence, consistent with a disk actively forming new planets (Teague et al. 2016).
A serendipitous find
While the researchers had found what they had been looking for, one CS line also showed something they did not expect. The line in question corresponds to a change in the molecule's rotational speed (J = 5 –4), and it showed clear ring-like structures in the outer regions of the disk, at a distance of 95 astronomical units from the central star and beyond. (The astronomical unit corresponds to the average distance of the Earth from the Sun.) In our own solar system, the dwarf planet Pluto has an average distance of 40 astronomical units from the Sun; at 95 astronomical units, we would be at the very inner rim of the hypothetical Oort cloud, home of comets and other assorted small bodies.
Recall that CS is the line for which the disk is transparent. As such, its light is likely to reflect larger physical structures affecting the whole disk – as opposed to ordinary CO, which is more likely to show pure surface effects.
The astronomers also saw another structure, even further out at 180 astronomical units from the central star, right at the edge of the TW Hydrae disk: a ring that was slightly brighter in the CS light than the surrounding regions.
Comparing notes: gas vs. dust
Teague et al. then included an additional kind of observation. In 2013, a group around John Debes of the Space Telescope Science Institute in Baltimore had observed the TW Hydrae disk using light emitted by the central star, then reflected by the dust particles in the disk. These observations had shown a gap at about the right distance as that now found by Teague and his colleagues (Debes et al. 2013).
Teague and his colleagues used a similar, but markedly more detailed image taken by another group of researchers, this one led by MPIA's Roy van Boekel (van Boekel et al. 2016). The image had been recorded using the newly commissioned instrument SPHERE at ESO's Very Large Telescope to take high-resolution infrared images of the TW Hydrae disk. The observations made use of some specific capabilities of SPHERE: they specialized in polarized light to separate reflected light from light emitted directly by the gas and dust, and used a technique called differential imaging to suppress observational artifacts due to the telescope.
The SPHERE image shows three ring-shape gaps in the disk. The outermost, at 95 astronomical units from the central star, corresponds closely to the gap detected by Teague and his colleagues. That the gap is visible in two different modes, in the light emitted by part of the disk's gas and the light reflected by the gas, is already a strong indication that this is a structural feature, not the consequence of chemical reactions or the like.
Modeling the disk
For a more definite answer, the researchers created a realistic model of the disk. Their work started with a smooth disk model, based on previous observations of the disk as well as on physical and chemical equilibrium conditions. To this smooth model, the researchers added small perturbations in the physical structure corresponding to the rings and gaps that had been observed.
They then computed how these perturbations would affect the disk: how, in various disk regions, temperatures would change, chemical abundances alter. Disk properties are closely tied with the radiation absorbed, reflected and emitted by the disk, so radiation properties had to be modeled, as well.
The researchers calculated matter and radiation properties for seven different models, with slight variations in the gap size and location. In some of the models, the gap is a feature of the dust only, while the gas density is unaffected.
Each of the seven models leads to a somewhat different appearance of the disk. Comparing these various models with the available observations, both for the CS radiation with ALMA and light reflected by the dust with SPHERE, a clear picture emerged.
The slightly enhanced ring at 180 astronomical units is indeed readily explained by the local state of the CS molecules – a thermodynamic effect: Near the edge of the disk, matter densities are very low. Radiation can reach more of the CS molecules attached to dust particles and make them evaporate. This slight increase in gaseous CS can explain the brighter outer ring.
But the gap at 95 astronomical units is indeed likely to be a region with very little gas and dust. Within the gap, the density is only about 45% as big as in the adjacent regions.
Three-dimensional structures at last
This is only the second time such density structures in a protoplanetary disk have been detected. Spectacular images showing rings and lumps have been around for a few years, but until late 2016, there was no such image where researchers could be sure these rings and lumps represented three-dimensional, physical structures. Instead, the features could have been explained by differences in dust grain properties – not necessarily density variations.
The first study that was able to confirm real density structures went on in parallel to the research of Teague and his colleagues: it was published by Andrea Isella and his team in December 2016 (Isella et al. 2016). That group used ALMA to detect light reflected by the dust, finding ring-like structures that were also visible in some specific CO lines.
The two analyses signal a new phase in the study of protoplanetary disks: From research based mostly on spectra, astronomers have graduated to detailed images in the years 2013 and following. Now they have taken the next step: images in different wavelengths that combine to yield information about the three-dimensional structures of such disks.
A far-out planet?
Having established that the gap at 95 astronomical units is a region of lower gas density, the logical next step is to look for possible causes. An attractive explanation is the presence of a planet. As the planet follows its orbit, it would attract gas and dust, sweeping its orbit and surrounding regions clear. (Within our Solar System, this is part of the definition of a planet: sufficient gravity to clear its orbit of smaller particles and debris.)
Given the properties of the model, Teague et al. are able to estimate the mass of such a hypothetical planet as between 12 and 35 times the mass of the Earth. A planet with smaller mass could only have produced a smaller, a more massive planet a larger gap than the one visible in the observations.
Far-out planet formation?
If there is no planet in the gap, then the gap could at least be an indication that there is a planet in the making: an instability that concentrates gas and dust in one region, and removes them from surrounding regions (creating the gap). Such an instability would provide good conditions for dust particles to clump, and clumps to grow, eventually forming a planet.
Both explanations, either the instability or the presence of a planet, would show that planet formation is possible at large distances from the central star. In the present models of planet formation, this requires some explanation: in these models, the only accepted way of forming far-out planets is in a much earlier evolutionary stage than that of the TW Hydrae disk, and in much more massive disks than this, which can become gravitationally unstable.
Future directions
The present results also suggest a direction for future observations. The immediate next step will involve more detailed observations of the TW Hydrae disk targeting specific additional molecular lines.
For instance, diazenylium ions (N2H+) are readily destroyed by CO, and thus will only exist in reasonably high abundances in regions where CO is frozen out. Thus, these ions are able to trace deep regions within the disk, which are sufficiently well shielded from stellar radiation.
Hydrogen cyanide molecules (HCN) on the other hand are particularly well suited to tracing a young planet that is still hot from collecting additional matter from its surrounding. The presence of such a planet should evaporate HCN from the surrounding dust, creating a tell-tale bright spot in HCN line observations that indicates the location of the planet.
Should these and similar observations confirm either the presence of a far-out planet or planet-formation at such great distances from the star in the TW Hydrae disk, the ball would be in the court of those researchers who attempt to find the general framework for planet formation around young stars – and who would need to explain how planet formation can happen so far out.