High-Mass Star Formation
How do the most massive stars in our and other Galaxies form? How do they shape their clustered environment as well as the interstellar medium (ISM)?
Physical and chemical conditions for high-mass star formation
High-mass stars (>8Msun) constitute one of the most important building blocks of our Galaxy and the universe as a whole. If measured by pure number counts, they only make up a very small fraction of all stars, however, their luminosities are so enormously high (they scale approximately with the third power of the mass), that massive stars dominate the energy budget of all forming stars. Through different physical processes they inject vast amounts of energy in their environment, e.g., during their birth by energetic jets, throughout the whole lifetime via their strong UV-radiation and at their death by the supernovae explosions. Furthermore, high-mass stars are the cauldrons where all the heavy elements are formed. Hence, without building heavy elements in the first place, no life as we know it would be possible at all. Thinking on larger spatial scales, one should keep in mind that massive stars are almost the only stars we can observe in extragalactic systems (low-mass stars are too weak at the given distances). Therefore, all stellar knowledge known for far away Galaxies is extrapolated by observing the most massive stars therein.
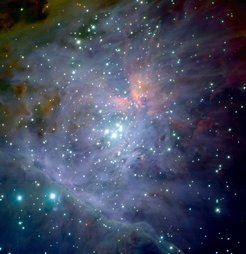
Although the importance of high-mass stars is undisputed, their actual formation processes are still poorly constrained. The main reason for that is the short formation time-scale because they start hydrogen burning before the accretion of gas has stopped. This implies that strong radiation pressure is put on the remaining infalling gas that can revert the infall in simple spherical models. To overcome these problems different scenarios are proposed, the main two are: (a) A scaled-up version of low-mass star formation where the gas accretes onto the protostar through an accretion disk with significantly enhanced accretion rates compared to their low-mass siblings. The second scenario (b) builds on the fact that high-mass stars always form in clusters and the whole cluster potential has to be taken into account. This picture is more dynamic and relies on the competitive accretion of the cluster members from a common envelope. In reality, a merged version of these two pictures may represent nature best. However, that is topic of current research. The high-mass star formation research at the MPIA addresses this field with a very diverse approach tackling various physical and chemical processes via different routes. On the one hand, we are following a multi-wavelength observational approach where various state-of-the art observatories are employed to investigate the plethora of physical/chemical processes (e.g., the Very Large Telescope (VLT), the Large Binocular Telescope (LBT), the Plateau de Bure Interferometer (PdBI, now NOEMA), the Submillimeter Array (SMA), the Atacama Large Millimeter Array (ALMA), the IRAM 30m telescope, APEX, Spitzer, Herschel. On the other hand, the theory group at the MPIA also models the collapse of the massive gas cores and the associated processes, e.g., the formation of accretion disks and outflows. In the following, we outline a few of the directions in more detail:
Galactic studies
Recent years have witnessed tremendous efforts in surveying our whole Galaxy - or at least large fractions - at many wavelengths. These surveys are crucial if one wants to study the properties of the gas, dust and stars on a Galactic scale. For example, studies based on the ATLASGAL and Spitzer surveys (GLIMPSE and MIPSGAL), allowed us to study the distribution of massive star formation regions within our Galaxy as well as the approximate time-scale of the earliest evolutionary stages, namely the high-mass starless cores. These Galactic plane surveys are treasure trunks for many years of science, and the existing surveys are complemented with the Galactic plane far-infrared survey conducted with Herschel (HIGAL). Analyzing these large datasets is a technical challenge but also promises great results.
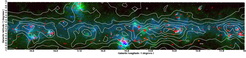
3-color image taken from Tackenberg et al. (2012). Red ATLASGAL, green 24μm, blue 8μm. Contours display CO emission from Dame et al. 2001)
At long radio wavelength, we are leading a large Galactic plane survey at cm wavelengths with the VLA. This survey THOR (The HI/OH/Radio recombination line survey of the Milky Way) addresses a plethora of scientific topics. Among them, we are studying the dynamics of the interstellar medium and cloud formation from the atomic to the molecular phase, and directly compare the results to theoretical cloud formation models. OH and HI absorption/emission line studies enable detailed investigations of the cold phases of the interstellar medium. Combining the data with Herschel/SOFIA C+ observations can reveal the nature of photodissociation regions. The HII region recombination lines allow us to study, e.g., the dynamics of potentially triggered star formation. The continuum data reveal spectral indices for approximately 10.000 sources, and we more than doubled the number of supernovae remnant candidates in the survey area. In addition to that, unexpected exciting results will emerge from such a novel survey.

THOR (HI/OH/Recombination line survey of the Milky Way) data: The top panel presents the integrated atomic HI gas emission whereas the bottom panel shows the 1.4GHz continuum emission that mainly stems from HII regions, supernova remnants and extragalactic background sources.
Employing the surveys of the Galactic plane as well as studies of nearby Galaxies allows us to set the properties of the interstellar medium (ISM) into a general context. For example, in a recent study of the nearby Galaxy NGC4321, our Milky Way disk and the central molecular zone of the Milky Way, velocity oscillations were found on all spatial scales and in the diverse environments. These results show that the structure of the ISM cannot be considered in isolation, but that is structure, formation and evolution is controlled by dynamical gas flow over orders of magnitude in spatial scales.
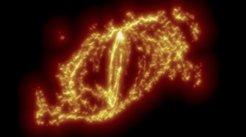
The initial conditions of high-mass star formation
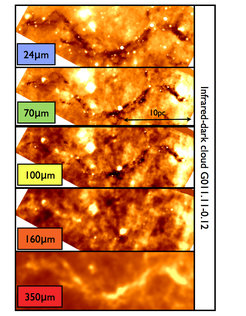
High-mass star formation begins (and proceeds for a significant evolutionary time) deeply embedded within their natal gas and dust clouds. Such regions are opaque in the optical and near-infrared wavelength and hence need to be observed closer to the peak of their spectral energy distribution at far-infrared and (sub)mm wavelengths. To characterize the initial conditions where high-mass star formation begins, our institute follows several projects utilizing single-dish (e.g., APEX, IRAM 30m), space-based (e.g., Herschel) as well as interferometric observatories (e.g., PdBI, now NOEMA). To highlight one project, within the guaranteed time Herschel program EPOS (The Early Phase Of Star formation) we studied 44 very young high-mass star-forming regions in great detail in all Herschel bands between 70 and 500 μm wavelengths. These data allow us to set tight constraints on the temperature and gas (column) density distribution which are crucial parameters for any model of high-mass star formation. Furthermore, these data are accompanied by spectral line observations to investigate the kinematic properties of these gas clumps.
Collapse and accretion disks
After the collapse of the initial gas clump has begun, it is unclear in which fashion it proceeds. For example, does the original gas clump early on fragment into many little gas cores that accrete from their common envelope? Or do larger-scale entities remain that collapse as a whole and form the massive star out of this restricted environment? Do real Keplerian accretion disks form at the centers of these regions? Do we always observe multiple systems? Many of these questions are best addressed with interferometers because small spatial scales need to be resolved. Therefore, we are studying with NOEMA (formerly PdBI), ALMA, the SMA and the VLA diverse types of high-mass star-forming regions from starless clumps to very hot molecular cores that host already embedded massive stars. For example, in almost all cases we find rotational motions of the central gas clumps, however, on scales between several 100 and 1000 AU and without Keplerian rotation signatures. These structures rather appear as larger-scale rotating torii that may host genuine Keplerian disks at their so far unresolved core centers.
We are conducting a large survey (CORE) of young high-mass star-forming regions at NOEMA resolving the fragmentation and rotational, potential disk properties at a spatial resolution of 0.2-0.3''. This CORE project adresses these fundamental topics from a statistical point of view for 20 regions. As a next step, then ALMA will be the instrument to resolve these structures at even higher spatial resolution (down to ~0.05'') to determine the nature of these objects on smallest spatial scales.
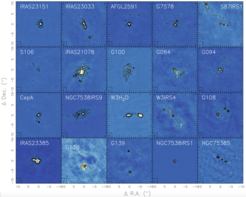
Millimeter continuum images of the NOEMA CORE project studying 20 high-mass star-forming regions.
Complementary to these observational approaches, the theory group investigates the collapse of high-mass gas clumps with numerical simulations, and they also find that 2-dimensional accretion disks have to form (e.g., Kuiper et al. 2011).
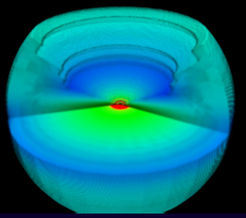
3D hydrodynamic simulations.
Jets and Outflows
A direct implication from the accretion-disk-accompagnied star formation in the presence of magnetic fields is that energetic jets and outflows are driven from the innermost regions of the protostars and disks. The physical properties of these outflows have been studied extensively over the last decade, but it remained unclear whether they are similar to their low-mass siblings, hence mainly magneto-hydrodynamically (MHD) driven, or whether the radiation is important here. To understand the physical processes better, we have studied numerically the outflow launching of MHD jets in the presence of radiation from the central object. The results clearly support the observational picture that early on MHD forces dominate but the more massive the star grows the more the radiative component becomes important. Hence, an interplay between magneto-hydrodynamic forces and radiation best explains the observations.
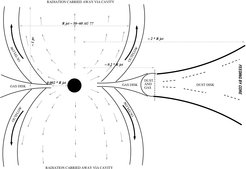
Clusters and cluster formation
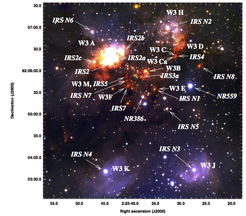
Since the formation time-scale of high-mass stars is very short (on the order of a few 100.00 years), quickly whole clusters form that can still harbor signatures from the initial formation processes. Imaging these very young clusters in the continuum as well as spectral lines allows us to study the stellar and gaseous components of young clusters. For example, we can spectrally classify many cluster members and by comparing these with evolutionary tracks determine the approximate ages of these young clusters. Setting them into context with simultaneously observed embedded protostars within the same regions, we find that star formation does not always proceed coeval but that within the same cluster several generations can form after each other. There exist also strong indications that the most massive stars form last during the cluster evolution.
Magnetic fields
Magnetic fields are among the most neglected properties in star formation. This is largely due to technical difficulties on the theory as well as observational side. Observing for example Zeeman and polarization effects is very challenging, and similarly conducting full-fledged magneto-hydrodynamic numerical simulations has been a tough task. However, in recent years the situation has changed considerable. While MHD simulations become regularly possible, in particular the observations of polarized emission from cold dust and even CO now allows us to study the magnetic field properties in much more detail than ever before. Studies indicate that on outflow scales the magnetic field appears to be aligned with the outflow. On smaller core scales, the data indicate in some cases hour-glass-like structures as predicted in the ambipolar diffusion picture. However, one should keep in mind that these studies are still in its infancy, but that the coming years, in particular with the considerably higher ALMA sensitivity, promise exciting new results.
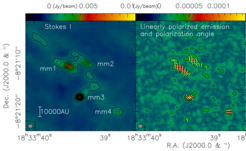