Disks and Extrasolar Planets
Protoplanetary disks around young stars are the birthplaces of planetary systems. Most of their mass is in the form of molecular hydrogen with small admixtures of solid particles. The initially micron-sized particles grow through mutual collisions to form larger meter-sized bodies with further growth potentially assisted by gravitational instabilities in dense dust layers.
At the end of this process rocky planets can form. If enough mass is available the central cores attract gas envelopes—a process that leads to the formation of giant planets. In the outer regions of massive disks, gravitational disk instabilities may trigger the formation of massive planets. The properties of gas-rich disks depend on the spectral type of the central star, the star-formation environment, and other parameters such as metallicity. Our research is establishing the connection between disk properties and the structure of planetary systems. We have searched for disks from relatively massive early B-type stars to brown dwarfs and were among the first to measure the disk masses of these very low-mass objects.
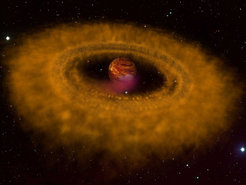
In the gas-rich protoplanetary disks, planets can migrate and open gaps if they are massive enough. Adaptive-optics assisted observations, interferometry from mid-infrared to (sub)millimetre wavelengths and observations with the Hubble Space Telescope (and soon with the James Webb Space Telescope) are providing the spatial resolution to search for gaps and other asymmetries in protoplanetary disks. The PSF Department is pursuing a multi-wavelength approach to characterize disks with a special emphasis on high-resolution techniques and spectroscopy. We have built dedicated instruments for ESO's Very Large Telescope Interferometer, JWST, and the planet finder SPHERE to characterize such disks.
The gas-rich disks are also chemical factories leading to the formation of water and even complex organic molecules. How water is delivered to Earth and rocky extrasolar planets is one of the most fascinating scientific questions we want to answer. Protoplanetary disks are members of the wider class of accretion disks transporting mass to the central star and angular moment to the outer disk regions. Viscosity produced by turbulent motion is the driver of these transport processes. After about 10 Myrs most of the disks lose their gas and enter the stage of debris disks where dust is produced by collisional processes among more massive bodies and a dynamical re-structuring of planetary systems can occur. Debris disks and their connection to exoplanets is another important research topic of our Department.