Molecular Cloud and Disk Chemistry
There is a wealth of molecules out in the Universe constituting the material of galaxies, stars, planets and everything in between. Surprisingly chemically rich, various cosmic environments reveal distinct degrees of chemical complexity that we can study via multi-wavelength observations of molecular emission lines.
Our group researches in fields of astrochemistry stretching over evolutionary stages of the interstellar medium from cold, dark clouds to protoplanetary disks and comets. Below you find a listing of ongoing or previous research projects in our group over the years.
"Chemistry In Disks" (CID) collaboration
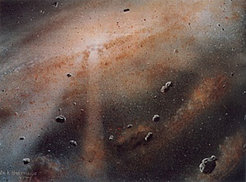
One of the most intriguing questions in astronomy is to understand conditions under which planets and their atmospheres form. Protoplanetary disks made of dust and gas are believed to be birthplaces of planetary systems, like our own Solar System. Observations of these objects in molecular lines and dust emission, analysis of meteoritic and cometary samples, and modeling their physics and chemistry are needed to unravel the initial conditions and chemical complexity at the verge of planet formation. The new observational facilities like ALMA, MOEMA, VLA, VLT (and JWST in the future) provide for the first time high angular resolution and sensitivity to perform such studies. Consequently, methods, models and tools to deal with a large amount of such high-quality observational data on disks have to be critically re-evaluated and adjusted.
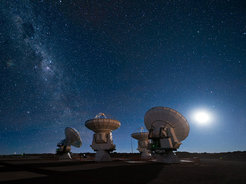
Therefore, our research interests aimed at studying in detail physical and chemical evolution of protoplanetary disks and the solar nebula, both theoretically and observationally. The main directions of research are:
(1) (sub-)millimeter observations of protoplanetary disks with modern radio telescopes coupled to the modeling of time-dependent evolution of disk physics, chemistry, and radiative transfer in lines of key molecular tracers,
(2) understanding the role of isotopic fractionation and its relevance to thermal/irradiation history and transport processes (with links to meteoritic science), and
(3) the genesis of complex molecules prior and during formation of planets (with links to cometary science). These disk studies are performed by our group in the framework of the large international collaboration called "Chemistry in Disks" (CID), which involves a dozen of scientists from Germany, France, USA, Hungary, etc.
Isotopic fraction in the interstellar medium and protoplanetary disks
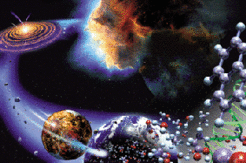
Probing the properties of the interstellar medium is essential in understanding the formation of stars and planets. In these stellar nurseries, stars and planets are formed through ongoing accretion of gas and dust until stars are formed, heating their surroundings and housing the birthplace of planets and other astronomical bodies. These nurseries are also the scene of an intense chemistry with sets the conditions for the continued evolution in the subsequent evolutionary stages. They are also characterized by a rich isotopic chemistry which is boosted by lack of destructive reactions in these cold environments, and the chemical abundances evolve from simple molecular hydrogen abundant in cold, dark clouds, to prebiotic amino-acids present on planets. The rich chemistry present in the evolution of the interstellar medium, supplies us with numerous ways of probing the interstellar medium and its properties. The modern high-sensitivity, high-resolution measurements call for better theoretical models in order to understand the results.
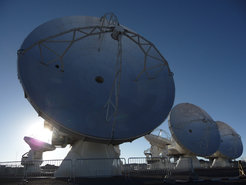
Isotopes have since long proven essential as probes of the interstellar medium by studying the relative abundances between species and their isotopes. For deuterium, the first isotope of hydrogen, cold, dark clouds are reckoned to be the scene of extreme molecular deuteration. Despite the cosmic D/H ratio determined to be ~10-5, many molecules show observed ratios well above this value, and some show ratios of unity or higher. The key player in this is H3+ and its deuterated isotopologues, which unlocks deuterium from its main reservoir HD, and distributes it in colder environments efficiently to other molecules
Our research is therefore aimed at investigating the chemical evolution of molecules containing isotopes of H, C and O in the context of planet and star formation. We study the isotope chemistry over evolutionary stages from dark clouds and prestellar cores to protoplanetary disks and comets. For this we developed an extensive chemistry network that includes a full set of deuterium isotopic chemistry and nuclear spin-state exchange processes.
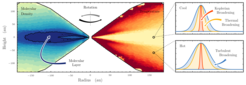
The scheme of the vertical disk structure (left panel). Shown are typical molecular abundance distribution (left cone) and gas temperature (right cone). For optically thin lines the local line profile is a combination of Keplerian rotation, thermal broadening, and turbulent broadening.
The turbulent motions in a protoplanetary disk will have profound effects on both the physical and chemical evolution of the disk and on the efficiency at which it produces planets. Such stirring motions will broaden the line profile of observed molecular tracers, providing us with a chance to measure the magnitude of the turbulence. Constraining the values is essential in developing our models of planet formation.
Recent advances in telescopes, particularly with the advent of ALMA, allow able to measure the linewidths to equisite precision. The total linewidth is a combination of turbulent, thermal and Keplerian broadening. Thus, in order to disentangle the turbulent component we must measure the temperature of the emitting gas extremely accurately and account for , using models and our understanding of the disk's rotation, the Keplerian broadening. Using line ratios or ensembles of molecular tracers, we are able to constrain the temperature and thus turbulent motions in a particular region of the disk.
ALMA is providing data rich in kinematical information which we are only beginning to understand, giving us an unparalleled view into the kinematics of a planet forming disk.
High-mass star formation
High-mass star-forming regions are among the richest molecular environments in space, in particular during their so-called "Hot Molecular Core" phase. During the birth of high-mass stars the central objects heat up their surrounding dense gas cores and a very active gas-phase and grain-surface chemistry gets into action. Millimeter and submillimeter spectra during that evolutionary phase show extraordinarily rich spectral line signatures of a large variety of molecules (see example spectrum in Figure below):

In the high-mass star formation group at the MPIA we are addressing this chemical complexity by a two-fold approach. On the one hand, we want to understand the chemical evolution during the formation of high-mass stars: These regions start of as early cold infrared dark gas clumps with a simple chemistry likely resembling that of the general ISM. They then start the ignition very early when they are still embedded in their natal gas clump. The central objects heat up the clumps as massive young stellar objects (also called high-mass protostellar objects) and the chemical composition becomes more complex until they form the hot molecular cores with the richest chemistry. Further on during their evolution, the central stars ionize the surrounding gas clumps from the inside-out (forming ultracompact HII regions) and the chemical complexity decreases again. Close collaboration between observers and theorists is very important.
Statistical studies of the chemical evolution in high-mass star formation
To characterize this evolution, it is mandatory to study statistically significant source samples within each evolutionary stage, determine their corresponding chemical abundances, and then model these with our in-house chemical networks. Led by PhD student Thomas Gerner, we investigated a sample of 60 high-mass star-forming regions from the initial starless core phase via high-mass protostellar objects to hot molecular cores and finally ultracompact HII regions. Figure below shows examples of the different evolutionary stages:
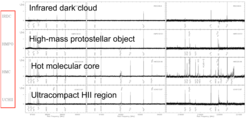
These data allow us to quantitatively evaluate the evolution of the chemical abundances during the different phases of star formation (see Fig. below). In addition to this characterization, we also model the evolution of typical massive star-forming complexes with state-of-the art chemical codes. Introducing varying physical conditions, in particular the temperature and density structures of the regions, we were able to model most of the measured abundances (Gerner et al. 2014).The tight collaboration between observers and theorists is key to the success of such projects.
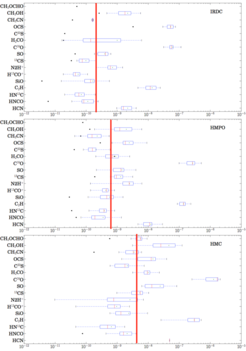
The deuteration of molecules is an aditional important chemical tool to characterize the physical processes of the gas because the deuteration inherits gas signatures at the earliest evolutionary stages and keeps them in their abundance profile. Therefore Gerner et al. (2015) studied the same source sample in 4 different deuterated species, and measured and modeled again the abundances. On average we find deuteration ratios between a few permille and a few percent. While for HNC, HCO+, and N2H+ the deuteration decreases with time, for HCN it increases during the evolution. This indicates that for some species deuteration does not only take place in the cold phase but that warmer deuteration pathes exist as well.
Chemical substructure of high-mass star formation complexes
Another important aspect of the chemistry during high-mass star formation is the complexity within each region. High-mass stars solely form within clusters, and these many stars do not just excite their immediate surrounding differently depending on the mass of each star, but the protostars also drive outflows that shock the gas in the environment and excite a different shock chemistry. Furthermore, disk should form that again evolve differently to the envelope gas. To differentiate these chemical sub-structures within individual regions interferometric high-spatial resolution studies are required.
Investigating the most famous hot molecular core Orion-KL, PhD student Siyi Feng dissected the chemistry of that region with combined data from the Submillimeter Array (SMA) and the IRAM 30m telescope (Feng et al. 2015). This combination allowed her to resolve the substructure of the region and at the same time measure the varying abundances in that region in great detail (Figure below shows a few example images).
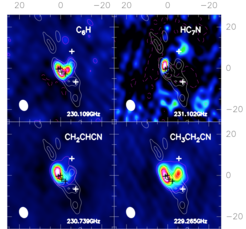
However, looking just at Orion-KL is not sufficient to understand the chemical substructure in such type of regions in a more general sense. Therefore, Feng et al. (subm.) extended these investigations to more regions, in particular the famous clustered high-mass disk candidate NGC7538S.This region resolves into three main subcores which all exhibit similar gas column densities measured by the continuum emission (Figure below, left panel). In contrast to that, the chemical signatures between all three cores vary enormously. While one core exhibits signatures of a typical hot molecular core, the second has already lower abundances, and the third core shows almost no line emission at all, which is indicative of very low chemical abundances. These differences show that the chemistry does not only evolve in a general sense during the formation of the whole large-scale gas clump (as outlined in the statistical study above), but that the chemistry varies on much smaller scales within each region. One of the major reasons for these variations appears to be different temperature evolutions of the various subcores (Feng et al. subm.).
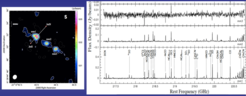
In summary, combining large-scale and small-scale spectroscopic studies of high-mass star-forming regions allows us to understand the chemical complexity during the formation of high-mass stars. Furthermore, these regions are ideal laboratories to search for complex molecules and precursors to life.