Laboratory Astrophysics
Our understanding of key astrophysical processes in the interstellar medium and circumstellar environments is based on the understanding of the physical and chemical processes that generate and modify circumstellar and interstellar dust grains and molecules under different conditions. They can be observed in nearly all astrophysical environments including evolved stars, star-forming regions, protoplanetary disks, comets, and atmospheres of exoplanets.
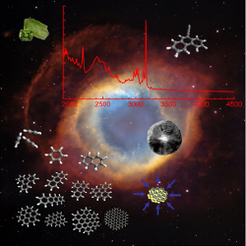
The MPIA runs a laboratory astrophysics facility at the Institute for Solid State Physics of the University of Jena and two new laboratories at the MPIA. In the Laboratory Astrophysics and Cluster Physics Group, we focus our research activities on two main areas: cosmic dust in its different forms and interstellar ices and the related chemistry leading to the formation of complex organic and even prebiotic molecules.
Nanometer- and micrometer-sized refractory dust grains are extremely important for the evolution of stars, galaxies, interstellar matter, and exoplanet atmospheres. Their optical properties dictate the interaction with photons and therefore the temperature of dusty regions. Formation mechanisms and radiation-induced processing of grains influence their internal structures, sizes, and shapes, and therefore, their spectral properties. Formation and radiation-induced modifications of cosmic dust grains occur at various conditions such as high temperatures in circumstellar shells around evolved stars or cryogenic temperatures in the interstellar medium. Laboratory methods including laser evaporation of solids and subsequent condensation in quenching gas atmospheres and laser pyrolysis of gases are used to simulate the high-temperature nucleation and condensation processes. The formation of dust at temperatures around 10 K can be simulated in ice matrices. Carbonaceous, siliceous, sulfide, and metal grains as well as astrophysically relevant molecules that may play a role as progenitors of grain formation are our main topics. Elaborated analytical tools comprising in-situ optical spectroscopy in the UV and IR range, mass spectrometry, chromatography, and electron microscopy at high and low resolution help us characterize the composition and structure of the produced laboratory particulate and molecular precursors.
A very recent research field in our group is Astrochemistry. New experiments designed for studying astrochemical reactions in interstellar ices covering cosmic grains such as carbon and silicates can simulate the physical and chemical conditions prevailing in molecular clouds and protoplanetary disks. We developed ultra-high vacuum (UHV) chambers equipped with closed-cycle cryostats, which can cool down ice or ice/dust mixtures to temperatures of less than 10 K. Energetic photons or reactive atoms produced by different sources can trigger reactions between molecular components in the ice. Chemical reactions are monitored by in situ IR spectroscopy and temperature-programmed desorption in combination with mass spectrometry. Complex organic and prebiotic molecules are formed in such ice mixtures clearly demonstrating their possible formation in the interstellar medium or protoplanetary disks under similar conditions. Grain surfaces can have a strong catalytic influence on reactions in ices or provide additional molecules by irradiation-induced erosion processes. Currently, a new UHV setup is being designed for studying astrochemical reactions in very complex molecular ices resulting in biological molecules such as carbohydrates and nitrogen-bearing molecules that are possible ‘precursors’ for RNA and therefore the origin of life. Reaction products will be laser-desorbed and in situ analyzed by high-resolution mass spectrometry.
Superfluid He droplets (T=0.37 K) provide an ideal environment to study reactions between embedded molecules and atoms under equilibrium conditions. Helium clusters can be produced by the supersonic expansion of pure helium gas at a pressure of 20 bar through a 5μm diameter pinhole nozzle cooled by liquid helium. The liquid He is able to absorb the energy of associative reactions, allowing the modeling of reactions on the surface of dust particles. In addition, the He droplet is an ideal nanocalorimeter, which is capable of detecting even small amounts of heat. In case of a chemical reaction the energy released during this reaction is transferred to the He droplet, causing the evaporation of He atoms. The evaporation of each He atom removes about 5 cm−1 of equivalent energy. The evaluation of a change of He droplet sizes after reactions provides an estimate of the amount of energy released. These data can be directly compared with the results of quantum chemical computations leading to unequivocal conclusions regarding the reaction pathways and the presence of energy barriers. The method was applied to study the reactions of C atoms with H2, O2, and C2H2.
Spectroscopy in the UV/Vis/IR is the link to astronomical observations. Therefore, a major part of our work is dedicated to the measurement of the spectral properties of dust grains and molecules. The spectral properties reflect the composition, structure, and temperature of the grains and molecules and have to be measured for every phase in their life cycle. Spectroscopy from the ultraviolet to the infrared of astrophysically relevant molecules can be performed at low temperatures in free jets and cryogenic ice matrices. Spectral properties of gas-phase condensed or processed dust materials can be measured in situ in a temperature range between 10 and 300 K.
Present projects in our group focus on: I) the formation and spectroscopy of real cometary materials consisting of dust grains, kerogen, and ice, II) the formation of carbonates in silicates and carbonaceous materials in different astrophysical environments and the related oxygen depletion by this process, III) the search for carriers of diffuse interstellar bands, IV) the formation of complex and prebiotic molecules in molecular ice layers, and V.) the X-ray-induced modification of ice-covered grains and formation of complex organic molecules ices at temperatures between 10 and 100 K.
For more information on our research, we refer to the website of the Laboratory Astrophysics and Cluster Physics Group in Jena and the "Origins of Life’’ Laboratory .